
2101 Smart Fusion
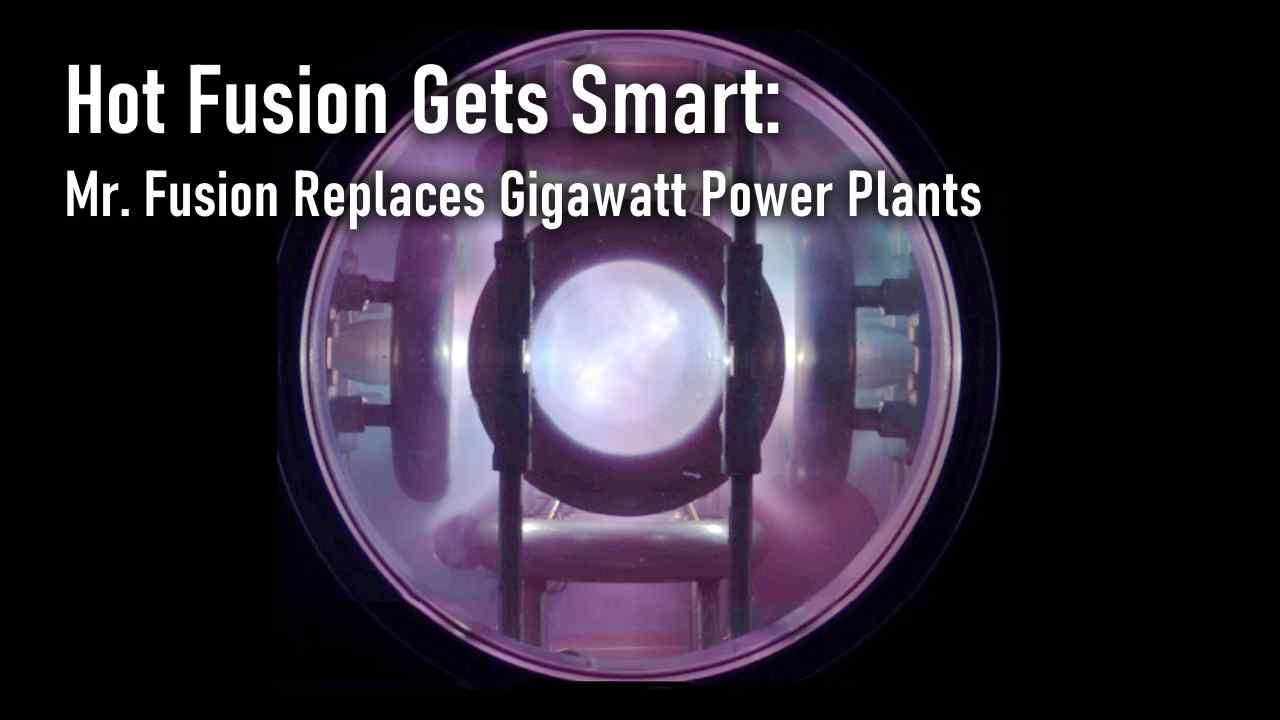
The history of nuclear fusion has its ups and downs, but above all it has taken a long time. New physical approaches lead to faster results than the large and expensive tokamaks. Decentralized fusion plants are replacing gigawatt power plants. Hot fusion is becoming smart.
For many decades, fusion research is dominated by the toroid approach. There are quick initial successes with tokamaks and stellarators. But by the 1980s at the latest, it becomes clear that the early promises cannot be kept. Nevertheless, a lot of money continues to be invested into huge fusion experiments.
While torus-oriented research drags on keeping generations of plasma physicists busy, a wave of new approaches to nuclear fusion emerges in the 2000s. The objective of classical tokamak research is to achieve fusion by generating hotter and longer plasma states. This is accomplished by subjecting the plasma to immense pressure and additional heating, resulting in temperatures reaching hundreds of millions of degrees. That is why it is called hot fusion.
Smart Fusion approaches differ from classical tokamak research in that they aim to provide the fusion fuel with precisely the required amount of energy to activate all of the plasma for fusion. These methods also utilize hot plasma, but some use fast ions produced by high voltage rather than heat, which is a more favorable physical process, thus earning the term "smart".
Although there are various smart fusion methods with distinct characteristics, they share a few fundamental differences from classical tokamaks. Specifically, they:
- enable neutron-free fusion processes and generate minimal radioactivity,
- produce electrical energy directly, eliminating the need for steam turbines,
- are more compact, cost-effective, and appropriate for decentralized energy production.
Despite being overshadowed by tokamak research for many years, smart fusion has made significant progress. Although flagship hot fusion projects receive 100 times more funding than smart fusion projects, smart fusion technology is proving to be more efficient. This is not only because the construction of large tokamaks takes a long time, but also due to the more favorable physical conditions that smart fusion methods provide from the start.
In the early 20s, several smart fusion projects achieve – what they misleadingly call – the break-even point in test reactors. These research reactors are still far from being real power plants. But the path is clear, and the objective is almost within reach. Ten years later, several fusion projects achieve break-even with fully operational systems.
After these successes, smart fusion is emerging from the shadow of tokamaks. Around 2035, various fusion companies are founded as spin-offs from scientific institutes and subsidiaries of energy companies. Now, large investments flow into smart fusion projects while the construction of the DEMO tokamak reactor as the successor to ITER is halted.
The world is still starving for energy and research reactors are being quickly transformed into demonstrators for commercial reactors. Around 2040, multiple reactors with power capacities ranging from 50 to 200 megawatts are becoming operational.
Most reactors use the pB11 process with protons and boron-11 ions, which produces no neutrons and thus little radioactivity. The fusion products are alpha particles, charged helium nuclei with high energy. They are converted directly into electricity by inverse cyclotrons and linacs eliminating the intermediate step via heat exchanger and steam turbine. The efficiency of electricity conversion is 80%.
The main technologies are:
- Electrostatic confinement with virtual anode (IEC).
- Self-focusing confinement using natural plasma instabilities (DPF)
- Colliding plasma rings in cylindrical confinement with reversed field configuration (FRC)
- Laser accelerator for protons with boron plasma target (LAF).
By mid-century, the first large commercial reactors with a capacity of 500 MW enter regular operation. Other technologies also enable smaller power plants with only 5 MW. Between 2050 and 2055, most patents fusion technologies expire allowing many new suppliers to enter the market. A vibrant service infrastructure for maintenance, operation, safety monitoring, and fuel emerges. Just 15 years later, around 2065, commercial fusion power plants already supply half of the base load.
In 2070, The Crash begins when power grids around the world fail, affecting all types of power stations, including fusion power plants. Whereas most types of power stations have a self-reliant mode, fusion power plants are unique in that they require a strong external power source to operate. They rely on technologies such as ion heating, strong magnetic fields, high-power lasers, cooled superconductors, and high-current discharges, all of which need a strong power source. While large wind turbines can produce ten megawatts without external power, a 10 MW fusion reactor requires nearly one megawatt of electricity to function. Therefore, converting fusion power plants to self-sufficient operation during a crisis is particularly challenging.
It takes many years for electricity from fusion power plants to be restored. The damage caused by the crisis and by the extended downtime must also be repaired, and the procurement of spare parts is extremely difficult. Fusion plants require highly specialized components such as superconductors, vacuum pumps, semiconductor lasers, nanometer-precise high-voltage anodes made of rare earths, and many other advanced components that are challenging to replace.
At the onset of the crisis, 60% of the power supply had already been converted to fusion, although the extent of this conversion varied significantly across different regions. In Europe, a strong emphasis had traditionally been placed on renewable energies and decentralization, which resulted in the prevalence of smaller plants that require less operating power. Eventually, the power supply from other reactivated power plants is used to power these smaller plants, enabling the fusion power plants to restart their operations. On the other hand, in the more industrialized regions of Africa and Asia, larger power plants and power plant complexes dominate. In some cases, the process of recommissioning these large plants takes up to 40 years.
In the early 22nd century, some countries and regions are still struggling to restore their advanced infrastructure, while others have already accumulated sufficient resources to improve upon fusion technology. Learning from The Crash, research is directed towards the development of smaller plants and decentralization. Soon 100 kW micro-scale fusion plants become commercially available. These micro-reactors are capable of powering individual homes and are about the size of a van. Although they have a lower energy density than internal combustion engines or old gas burners, they have the advantage of being able to operate for years on a hydrogen boron-11 cartridge producing only harmless helium gas as waste. Unlike fossil fuel power plants, fusion generators offer a clean and sustainable source of energy.
By the year 2120, as the global economy returns to pre-crisis levels, smart fusion technology provides 80% of the world's electricity production. Energy demand is expected to double every 15 years. To meet this growing demand, large gigawatt plants are being built to supply the energy for heavy industries like resource refining and waste recycling. They are supplemented by countless micro-reactors powering individual homes in a decentralized power grid. With energy no longer being a bottleneck to growth and prosperity, the future looks bright for the world.
The Great Expansion
There are still lots of small an short entries. The are great ideas and great milestones. They deserve more detail and love. First results of the expansion campaign:
2136 1000 in Space
2154 New Living Space
2155 Asteroid Mining
2158 Space Patrol
2179 Private Asteroid Base
2182 End of Venus Terraforming
2187 Moon Deportations
2192 Anti-Expansionist Terror
2197 Lunar Revolt
2205 Corporate Cosmos
2222 Space Piracy
2231 United Planets
2234 Orbit's End
2247 Quantum Leap
2248 Gemini Disaster
2269 1 Million in Space
2284 Great Separation
2291 Illegal Research
2293 Expansionist Uprising
2302 Solar Coalition
2303 Space Trader Coup
2312 Alien Spaceship
2318 Earth Union
2321 Earth Isolation
2326 No Interplanetary War
2333 Metric Impulse Drive
2337 Lunar Takeover